Synthetic polymers have contributed to many innovations in all aspects of modern life. Significant progress has been made in synthetic methods to obtain functional polymers, in the fabrication of polymeric nanostructures and in the fundamental understanding of their physicochemical properties. However, compared to the properties and functions of nature’s macromolecules, even the most sophisticated synthetic polymers still appear to be simple and only offer comparably basic functionality. Proteins are fascinating macromolecules, particularly from a polymer chemist’s point of view. The vast variety of functions that proteins can fulfill is not seen in any synthetic material. Enzymes for example act as catalysts, while other proteins fluoresce or control transport across cell membranes. Moreover, certain proteins can self-assemble into nanocontainers and nanoreactors. All these functions are essential molecular mechanisms that enable life and that render living tissue responsive and adaptive.
Our research encompasses an interdisciplinary, bio-inspired approach that combines polymer chemistry and protein engineering to create new opportunities for the sustainable synthesis of polymers and to design, engineer and realize materials and nanosytems with unprecedented new functions. Examples are the use of enzymes as catalysts for atom transfer radical polymerizations, the use of biocatalysis for malaria diagnostics, to develop polymersome- and protein-based nanoreactors for enzymatic reactions, and to use proteins as force-responsive sensor molecules in fiber-reinforced composite materials.
Some of our research highlights are presented below.
Biocatalytic ATRP
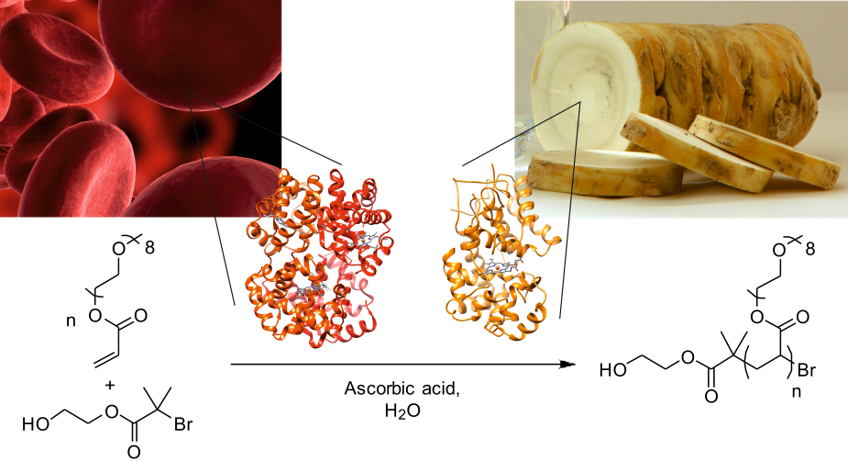
Controlled radical polymerizations such as atom transfer radical polymerization (ATRP) have revolutionized and revitalized the field of synthetic polymer chemistry over the past two decades. The transition metal catalysts required for ATRP, however, represent a drawback, since they can be toxic and environmentally unfavorable. A sustainable approach to catalysis involves the use of enzymes for numerous reasons: Enzymes are derived from renewable resources, most are non-toxic, function under very mild reaction conditions and often possess high catalytic efficiency and selectivity. We have discovered that metal-containing enzymes such as horseradish peroxidase, hemoglobin and laccase are able to catalyze ATRP. Biocatalytic ATRP (bioATRP) is the first evidence that enzymes can control radical polymerizations. Moreover, this “ATRPase activity” represents a previously unknown enzymatic activity of these promiscuous proteins. Apart from deciphering fundamental aspects of ATRPase activity, the Bruns group is constantly searching for those applications in which biocatalysts can outperform conventional ATRP catalysts. For example, laccase allows the controlled radical polymerization of N-vinylimidazole, an industrially relevant monomer that cannot be polymerized by classic ATRP in a controlled way because it strongly complexes copper ions. In contrast, the copper centers of laccase are within the enzyme, so that poly(N-vinylimidazole) is synthesized with low dispersities and predetermined molecular weights. Enzymes can also be separated easily from polymer products due to their different physicochemical properties, opening new routes to metal-free polymers, which are essential for many biomedical applications and for energy materials.
Polymerization-amplified malaria diagnostics


We have discovered a novel method to detect the presence of malaria parasites in blood samples. In collaboration with the Swiss Tropical and Public Health Institute (SwissTPH) and the Department of Medicine of the University of Fribourg, we investigate hemozoin as a catalyst for bioATRP. Hemozoin is generated by the malaria parasite when it digests hemoglobin. Hemozoin is used to catalyze a polymerization that leads to precipitation of the polymer at a temperature above 33 °C. The polymer solution passes from a transparent state to a cloudy one, and the speed of the reaction is directly correlated to the concentration of the malarial biomarker. Furthermore, very small amounts of hemozoin are enough to kick start a reaction.
The results of this study, published in the journal Nature Communications, have been implemented as part of an application project at AMI. Led by Dr. Jonas Pollard, the Hemolytics team is currently establishing a diagnostic method for malaria. This new tool could lead to more sensitive parasite detection, and help reduce healthcare costs. The test is specifically designed to discover asymptomatic carriers at risk of transmitting the disease, who could hinder complete eradication of malaria.
Light-responsive polymersome nanoreactors

Many biochemical processes in nature rely on a system being pushed out of equilibrium and then returning to its resting state automatically. An example is the light receptors in our eyes. On the molecular basis of light perception, a light-responsive molecule within these receptors isomerizes upon irradiation with light. At the same time, an enzymatic reaction converts the light-responsive molecule back to its initial state. If this would not be the case, the light receptor cells would not revert back to their dark resting state and would not be able to sense light repeatedly. Instead, an image would be engraved into our eyes like into a photographic film.
In an effort to create light-responsive catalytic nanosystems, we have prepared nanoreactors that react to light in a similar way. When irradiated with visible light, they are switched on. However, they only stay in their on-state as long as light is present. In the dark, they automatically revert back to their resting state in which their catalytic activity is switched off. The light-responsive nanoreactors were prepared by coupling a new kind of light-responsive molecules, Donor-Acceptor Stenhouse Adducts (DASAs), to block copolymers and allowing the polymers to self-assemble into polymersomes, i.e. hollow polymer nanocontainers. By conducting the self-assembly in the presence of enzymes, they are encapsulated in the polymersomes. DASAs were synthesized that absorb light of various color, so that nanoreactors could be switched by irradiation with either white, red or with green light. By using more than one enzyme, these nanoreactors allowed to control and orchestrate enzymatic cascade reactions by the different colored light in the same water solution. Indeed, it could be envisioned that by computing a sequence of light colors, different chemical products could be obtained in a predictable manner.
Such biocatalytic, light-responsive polymersomes could be used to produce drugs on demand, e.g. within a cell, or to release active compounds such as drugs in a fine-tuned, light-guided way. Moreover, our collaboration partners in this project, the group of Dr. Luciano Boesel at Empa, St. Gallen, aim to implement these nanoreactors in light-switchable plasters for drug delivery through the skin, which would enable a non-invasive delivery method of essential drugs to prematurely born neonates.
Bio-inspired materials: Force-responsive composites
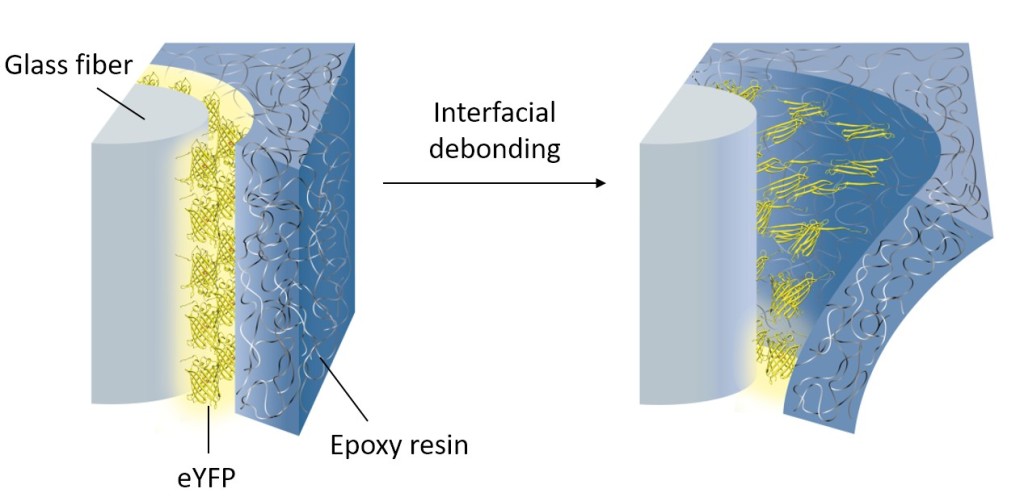
Mechanical forces and mechanical stimuli are an inherent part of nature, but their effect in controlling biochemical events has only recently been addressed in detail. Mechanobiochemistry is not only of relevance to understand processes in living systems, but it can also be a source of inspiration for the molecular design of novel materials with advanced properties. We have pioneered the force-induced modulation of photophysical properties of fluorescent proteins in polymeric materials in order to create materials that can self-report damage. For example, fluorescent proteins were conjugated onto glass and carbon fibers. These fibers were embedded into epoxy resin. The resulting fiber-reinforced composites could detect low velocity impact damage by a loss of fluorescent at the damage site. This allowed to visualize micron-scale damage such as fiber fractures and debonding at the interface of fibers and polymer matrix. The self-reporting system could find application as safety feature to detect barely visible impact damage in load-bearing composites or as analytical tool to monitor and characterize microscopic damage propagation in composites. Conceptually, the results demonstrate that mechanical forces can be transduced from materials to proteins if the proteins reside at an interface within the material, thus allowing to harness mechanically induced biochemical responses in a materials context.